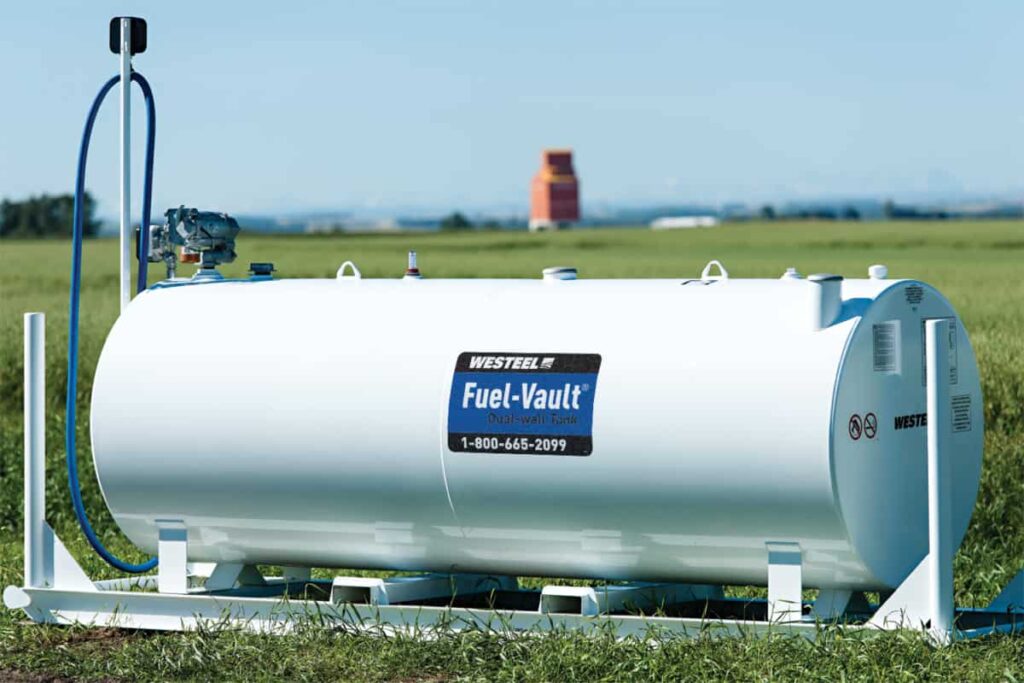
There are two primary fuels in use in aviation: avgas and Jet-A. They are similar in nature to automotive fuel (unleaded gasoline) and diesel, respectively, but have some key differences in both their properties and ability to be used in aviation applications. So, what is the difference between avgas, jet fuel, diesel, and auto fuel?
Avgas, auto fuel, and diesel are designed for piston engines, whereas jet fuel is designed for turbine engines. Avgas and jet fuel are formulated for the specific operating conditions and engine performance demands associated with aviation.
These fuels are all different in the types of engines they are designed for and the combustion and ambient temperature ranges in which they are designed to operate. Knowing the difference between these fuels is important not only for the safety of flight, but it’s also a pretty common question your non-aviation friends will ask you. In this post we’ll give you all of the information you need to know to give a solid answer to anyone who wants to know the differences between all of these fuels.
What is the difference between avgas, jet fuel, auto fuel, and diesel?
In order to understand the specific differences of these fuels, we need to first discuss the two main types of fuel. There are effectively two different types of fuel in use in combustion engines, “compression fuels” and “ignition fuels”.
Compression fuels, for the purposes of this discussion are those that require highly compressed fuel air mixtures in order to burn properly, and ignition fuels are those that are ignited using some type of spark or other ignition source.
Compression fuels are Jet-A (and its equivalents), diesel and kerosene. Ignition fuels are aviation (AvGas) and automotive (MoGas) gasolines.
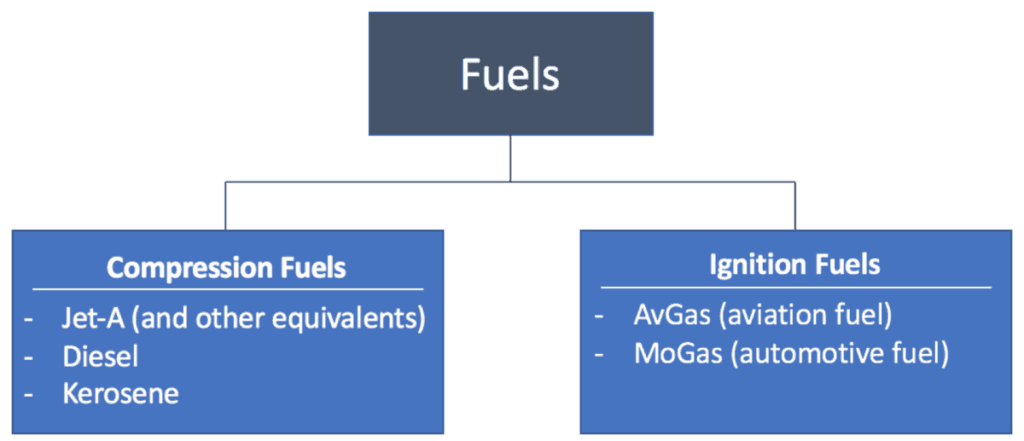
What Differentiates Compression Fuels and Ignition Fuels?
Ignition fuels and compression fuels are differentiated by a host of material properties that are a result of the refining process. Refer to “Why Does Jet A Fuel Cost Less than 100LL (Avgas) Fuel?” for additional details on the refining process.
Ignition Fuels
Simply put, ignition fuels are a mixture of lighter, more volatile compounds that require a specific ignition source to burn controllably.
Because ignition fuels are created later in the refining process, the carbon compounds that make up the fuel are more consistent and simultaneously more energetic and less stable. Ignition fuels will tend to ignite unevenly under sufficient pressure, a condition known as knock or detonation, which can quickly lead to engine damage.
Gasolines are considered ignition fuels, and require a spark plug to initiate the combustion process.
Compression Fuels
Compression fuels on the other hand are created earlier in the refining process and contain a wider variety of carbon chains that are more stable under a wider range of temperatures and pressures.
Additionally, because the auto ignition temperature for these fuels is lower, the associated pressures required inside combustion engines are lower, resulting in simpler engine designs.
Diesel, Kerosene, and Jet-A are all examples of such fuels. Compression of the fuel is used to initiate the combustion process eliminating the need for full time ignition systems within the engines that use these fuels.
What types of engines use each type of fuel?
Combustion engines come in two “flavors:” piston and turbine. Piston engines use the familiar cylinder and piston arrangement and use either two or four piston strokes per ignition event. Turbine engines continuously compress air, ignite it within a static combustion chamber and expel the exhaust product through an expansion turbine to drive the intake compressor.
Aircraft apply these engines differently depending on a range of design factors and requirements. Large modern aircraft generally use turbines and piston engines are favored by small personal aircraft.
Types of Fuel for Turbine Engines
Turbine engines use compression fuels, and in aviation these engines (which can be Turbojets, Turbofans, Turboshafts, or Turboprops – the difference between these is a function of what types of machinery are driven by the expansion turbine) all run on Jet-A and its military and international equivalents.
Some aircraft permit the use of aviation gasoline as an emergency fuel, but such operations are very strictly limited and induce a significant reduction in the operating life of the engine.
Turbine engines are designed around the use of Jet-A because of the fuel’s design to be highly stable and predictable in a wide range of environments, particularly at extreme temperatures and its high energy density.
Jet-A is also safer to handle in large quantities as a result of its much higher flashpoint, and when dealing with large quantities of fuel, it is beneficial to utilize a fuel that will not ignite easily outside of the engine combustion chamber. Commercial and military operations benefit massively from the reduced storage precautions required to store vast quantities of liquid fuel. As a result the broader turbine engine world has standardized around this type of fuel.
Types of Fuel for Piston Engines
Piston engines are the second category of aviation engine in common use, and depending on their design may use ignition or compression fuels. Piston engines may burn automotive or aviation gasoline (ignition fuels, though few can use automotive fuel… more on that later), or for aviation diesel engines, Jet-A (compression fuel).
The standard fuel used in aviation piston engines is aviation gasoline, or AvGas. AvGas as a technical term covers a wide range of different types of aviation fuel, but the most commonly used avgas is 100LL (“One Hundred Low Lead”).
The Experimental Aviation Association (“EAA”) has recently promoted inexpensive STCs (supplemental type certificates) allowing the use of alcohol free automotive fuel in certain aviation piston engines. The approved engines typically are approved for the use of lower octane fuels that are no longer produced specifically for aviation engines.
These STCs are popular with the owners of older light aircraft and with homebuilders and it offers a potentially lower cost source of fuel.
The rarest piston fuel is by far Jet-A. Aviation diesel engines are an abnormality in the piston aircraft fleet, as the technical challenges associated with building lightweight aircraft diesel engines are substantial.
What are the differences between compression fuels?
Kerosene is a generic name for a wide range of fuel products, of which Jet-A is a tightly standardized version. As a fuel product, Kerosene uses a wider range of quality and chemical property standards, which is acceptable for most terrestrial and marine applications. Because aviation requires consistent fuel performance across multiple engines and airframes and in rapidly changing environments, Jet-A is more precisely refined to remove impurities and ensure consistent performance.
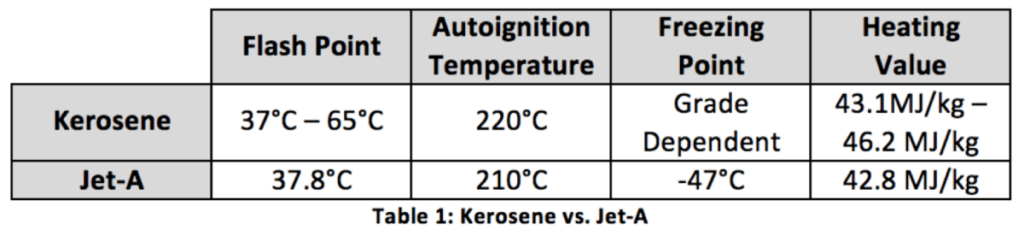
It is apparent from the table above that Jet-A is refined to much tighter tolerances. This is necessary to ensure the fuel meets the necessary performance across the operating envelope of turbine aircraft.
Commercial transport aircraft operate in cruise at temperature well below -47°C (fuel / oil heat exchangers raise the fuel temperature – see “How Does Fuel Aircraft Fuel Not Freeze During Flight?” or watch the 2-minute version below), making consistent thermal properties mandatory for Jet-A fuel. The slightly lower autoignition temperature allows for more reliable performance within the combustion chamber of the turbine engine itself.
Finally, the thermodynamic output of the fuel must also be consistent in order to ensure that flight planning manuals provide accurate data, as lower heating values produce lower thrust output, and reduced cruise performance. The criticality of consistent performance drives these tighter tolerances. While a typical aircraft turbine would burn generic kerosene, engine performance would be reduced and unpredictable.
The difference between diesel fuel and Jet-A is similar to that between Jet-A and kerosene, but of an even greater magnitude. Diesel fuel is designed and refined for use in diesel piston engines; it contains higher concentrations of impurities, and does not tolerate the wide temperature extremes needed in aviation.
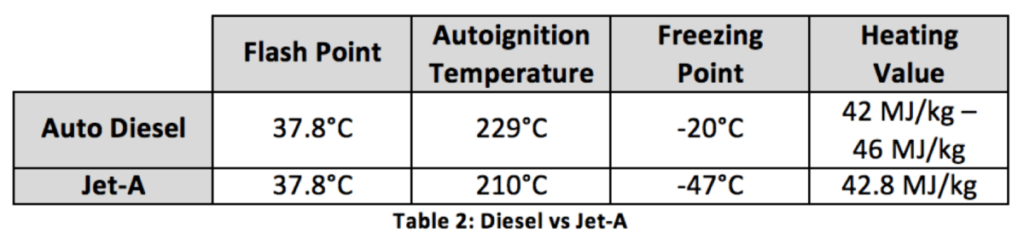
Reviewing the data in Error! Reference source not found. shows that the autoignition and freezing points of automotive diesel are substantially different than those of Jet-A. These differences are tied to the chemical makeup of the two fuels, with Jet-A being refined at higher temperatures, thus removing the sulfur compounds and providing a more uniform set of carbon molecules.
Jet-A is essentially an extremely high-quality diesel fuel, and indeed running a typical automotive diesel engine on Jet-A is likely possible.
However, the chemical compounds within diesel fuel have lubricating properties that ensure proper operating of fuel injectors, pumps and other mechanical components; the higher chemical purity of Jet-A strips away these lubricating compounds, resulting in more consistent performance, and a wider useful temperature envelope, but poorer mechanical properties.
Turbine engines would function using diesel fuel, but at the cost of reduced performance and the buildup of harmful deposits within the engine’s fuel system.
Additionally the higher vapor pressure of diesel fuel may interfere with proper atomization of the fuel within the combustion chamber leading to a host of other issues. There are turbine engines designed to use diesel fuel, but these engine have distinct design requirements from aviation turbines.
What are the differences between ignition fuels?
The common “ignition fuels” in use in aviation are AvGas and Auto Fuel (MoGas). The base chemical components of these fuels are virtually identical, as both are fundamentally gasoline and are extracted from the refining process at the same point. The difference between MoGas and AvGas is essentially a matter of a particular additive: Lead.
AvGas contains tetraethyl lead (TEL), to improve engine component lubrication, and boost the octane rating of the fuel. This type of octane boosting was common in automotive engines until 1975, when environmental concerns surrounding lead contamination resulted in the phase-out of lead in automotive gas.
Octane rating of the fuel is a measure of the fuel’s resistance to detonation (known as knock in automotive engines). Detonation is an operating condition in which the fuel ignites spontaneously under compression prior to normal firing of the spark plug. This results in severe pressure and temperature spikes within the engine, and quickly leads to severe engine damage.
Tetraethyl lead’s function as an octane booster is intended to allow higher engine performance at high power setting, particularly with the use of forced induction (superchargers and turbochargers). Tetraethyl Lead reduces wear in valve assemblies with the engine. The build-up of lead on valve guides and seats helps to lengthen the service life of these components.
A wide range of aviation engines are compatible with unleaded fuels such as MoGas, presuming the engine manufacturer has approved the use of unleaded fuel and the associated octane rating is met. Typically, these engines have fairly low horsepower outputs and low compression ratios, as such the aircraft they are installed in are typically small, lightweight aircraft.
The greatest hazard for aircraft engines using unleaded fuel is typically engine knock during climb at high altitudes, where the fuel air mixture is generally leaned to maximize engine performance, and where engine temperatures are highest.
Additionally, MoGas when compared with AvGas, reflects many of the same quality standard variability issues as Kerosene and Jet-A. The tighter quality control processes required for aviation fuels ensures that greater uniformity is present within AvGas.
One example of this is the use of ethanol as a fuel additive. Ethanol is increasingly mixed with automotive fuel to raise the octane rating and improve fuel economy at reduced cost to fuel refiners, but the precise mixture ratio is rarely placarded sufficiently to ensure safe aviation use.
Unfortunately, ethanol is incompatible with nearly all aviation piston engines and their fuel systems. The rubberized components used in aircraft fuel systems, from tanks, to hoses to seals are not compatible with ethanol and quickly deteriorate when ethanol laced fuel is used.
Where MoGas is used in aviation engines, it is ethanol free and the octane rating is typically equivalent to or higher than the “premium” fuel found at the local gas station in order to meet the minimum octane requirements of aviation engines.
Automotive engines have been designed to utilize a wide range of fuel qualities and tolerate a wide variety of additives to improve engine life and durability. The effect of these additives is numerous, but in particular, these additional chemicals raise the vapor pressure of MoGas, meaning that it evaporates from liquid to gaseous at a higher rate relative to AvGas.
This higher evaporation rate makes MoGas susceptible to vapor lock at high altitudes and at high temperatures, making engine starts more difficult and engine failures at low power settings more likely.
It is common for MoGas STCs to specify the mixing of certain quantities of AvGas to improve the vapor pressure, or to artificially cap the aircraft’s ceiling when using MoGas.
Quick Break: Have you subscribed to the Airplane Academy YouTube channel yet? Each week we’re putting out videos sharing aviation adventures, ride-along’s, answers to commonly asked training questions, and much more! Check it out here.
Related Questions
Why aren’t more diesel engines used in aviation?
Piston engine powered aircraft continue to rely on AvGas as a fuel source because the design requirements of diesel engines incur an inherent weight penalty, and new engine development is expensive and prolonged.
Diesel engines are typically heavier than their gasoline equivalents to meet the strength requirements associated with compression engine cycles. Automotive and aviation diesels alike nearly exclusively require turbocharging to be effective power plants as throttle response is a primary design consideration in both industries.
Additionally, aviation diesels are, primarily, derived from automotive diesels and thus must be geared down to drive a propeller. These gearboxes are failure prone, and often result in expensive replacement costs over dramatically shorter time intervals than is typical for gasoline engines. These additional mechanical systems drive up the weight and complexity of the engine, a serious detriment to the implementation of diesel engines as a primary powerplant for piston powered aircraft.
This resultant increase in weight generally results in an engine that develops less power for the same basic engine size and weight, resulting in lower aircraft performance.
Lower performance is not a detriment for certain segments of the aviation industry, particularly in the training sector and Asian and African markets, but sufficient sales have not yet materialized to justify the development costs and timelines necessary to certify new piston aircraft under current regulatory burdens.
The automotive roots of these engines do provide some benefits however, much of the research and development costs of the engine itself have been amortized by the automotive industry, and the engine control systems are typically electronic (i.e. FADEC controlled just like many turbine engines).
Electronic controls simplify engine operation and allow for extremely efficient operations. However, there is not yet sufficient net operating cost benefit for these engines to make significant sales gains within the piston aircraft fleet, either with OEMs or as aftermarket STCs.
Why do aviation piston engines need such a high octane rating?
Consider briefly your car’s engine. When you drive you spend little time with the throttle wide open developing maximum power. Additionally, your car’s ECU (engine control unit) is effectively a FADEC (full authority digital engine control) carefully controlling timing, fuel delivery and spark to eliminate nearly all knock, or detonation.
Now consider the typical aviation engine, which is designed to operate at or above 75% maximum continuous power for the entirety of its lifecycle, without the aid of electronic controls. It is apparent that if typical automotive engines were operated similarly to aviation piston engines, higher octane values would be required to maintain reliability.
The high octane rating requirements of aviation piston engines stem from the pinnacle of piston engine development in the 1940s into the 1950s. Supercharging and turbocharging drove the outputs of piston engines to extreme heights in order to maximize engine performance for military and transport aircraft.
The extreme operating pressures and temperatures required fuel that would resist detonation under high engine loads for hours at a time.
The requirement for aviation piston engines to maximize power output under continuous high load is the driving design requirement for the use of high octane rating fuels. While some aircraft engines are capable of operating on lower octanes, “practical” aircraft that utilize high performance or turbocharged engines are still limited to using high octane fuels to avoid fuel detonation.
Why can’t turbine engines use AvGas?
Turbine powered aircraft operate at environmental extremes that necessitate extremely stable, pure fuels that produce consistent, predictable thermodynamic performance. It is entirely reasonable to expect an aircraft operating in Dubai on a 50°C flight line to climb to cruising altitudes where the air temperature is as low as -60°C or perhaps colder.
As discussed previously, the chemical properties and ease of storage of Jet-A and its equivalents make it ideally suited to turbine engine operations. AvGas in contrast to Jet-A is contaminated with lead and has a dramatically higher vapor pressure; both of these factors make it difficult to use in high temperature environments.
During combustion, lead is deposited on combustion liners and expansion turbine blades, reducing efficiency aerodynamic efficiency of the turbine and increasing the engine temperatures, resulting in decreased engine life. The vapor pressure issue results in cavitation and vapor locking of fuel pumps. Anyone who has tried to start a hot turbocharged, fuel injected piston aircraft engine can attest to the difficulty of dealing with vapor lock in a fuel system.
Some turbine engines do permit operation with AvGas as an emergency fuel source. Note, that AvGas is an emergency fuel only. Steep limitations and impacts to engine service life accompany the use of AvGas as a turbine fuel if it is permitted at all.
As an example, the King Air 350 PT6A-67A engines permit 150 hours of AvGas usage over the course of multiple thousands of hours of engine service life. Additional limitations are placed on fuel pump operation and maximum altitudes.
While turbine engines will burn just about anything as a fuel, the impact to engine operations is significant if AvGas is used.
Are there Jet-A alternatives?
The primary operating challenges associated with Jet-A fuel revolve around low temperatures. There are two primary alternative fuels that help to lower the freezing point of jet fuel: Jet-B and TS-1.
Jet-B is a 30% Kerosene, 70% Gasoline mixture that is limited to use in very cold environments. The high vapor pressure of the gasoline component makes this a highly flammable fuel in warm environments. The gasoline component also lowers the freezing point to -60°C.
TS-1 is a Russian specification of fuel that similarly has a lower freezing point of -50°C again at the sacrifice of warm temperature volatility, with a flashpoint of 28°C.
Other jet fuel alternatives include an increasingly diverse set of synthetic and biologically derived fuels.
Widespread adoption of these fuels has yet to occur, though militaries and commercial operators have been experimenting with these types of fuels for well over a decade. Pure synthetic or biofuel fuel loads are not yet approved, with the highest mixing ratio of Jet-A to bio or synthetic fuel being 50%. Additional development work continues on these types of fuel.
Are there AvGas alternatives?
The environmental impact of lead produced by piston aviation and the shrinking supply base of 100LL producers and the sole source of tetraethyl lead has put 100LL at risk of extinction by logistics or by regulation.
There are multiple efforts to develop lead free high octane replacement gasoline fuels for piston driven aircraft. The FAA is evaluating a handful of candidate fuels in an array of aircraft engines and aircraft types.
The 93UL, UL94, UL102, and G100UL are all potential candidates to meet replacement requirements for 100LL. The primary challenges with these fuels are various: the increased cost, reduced energy density, toxicity, volatility, and equipment compatibility. Development testing and certification testing is ongoing with the ultimate goal of standardization on one or two of these fuels for use in piston driven aircraft.
Conclusion
The differences between AvGas, MoGas, Diesel, Jet-A and Kerosene, are both subtle and dramatic. AvGas and MoGas both require spark ignition engines to be used effectively with internal combustion engines. This is a result of their higher ignition temperatures and higher vapor pressures require careful engine management and chemical additives to manage engine knock or detonation.
In contrast Diesel, Jet-A and Kerosene, are burned using compression ignition engines. These fuels are all much more stable at higher temperatures and are far less susceptible to generating engine knock. The refining process differentiates these different fuel types both for purity and for chemical makeup.
While there is some overlap in the use of these fuels across engine types, by in large they serve to power specific types of engines, with gasolines powering piston engines and the Kerosene based fuels powering turbine engines.
Ultimately, AvGas and Jet-A have become standard fuels for the aviation industry as they meet stringent quality and performance metrics that ensure consistent high performance for any engine designed to use these fuels. As with most of aviation, the mission requirements and planned operating environments play significant roles in defining the engine and thus fuel for to be used in a specific aircraft.
While you’re on the subject… here are other articles on Airplane Academy related to fuel: