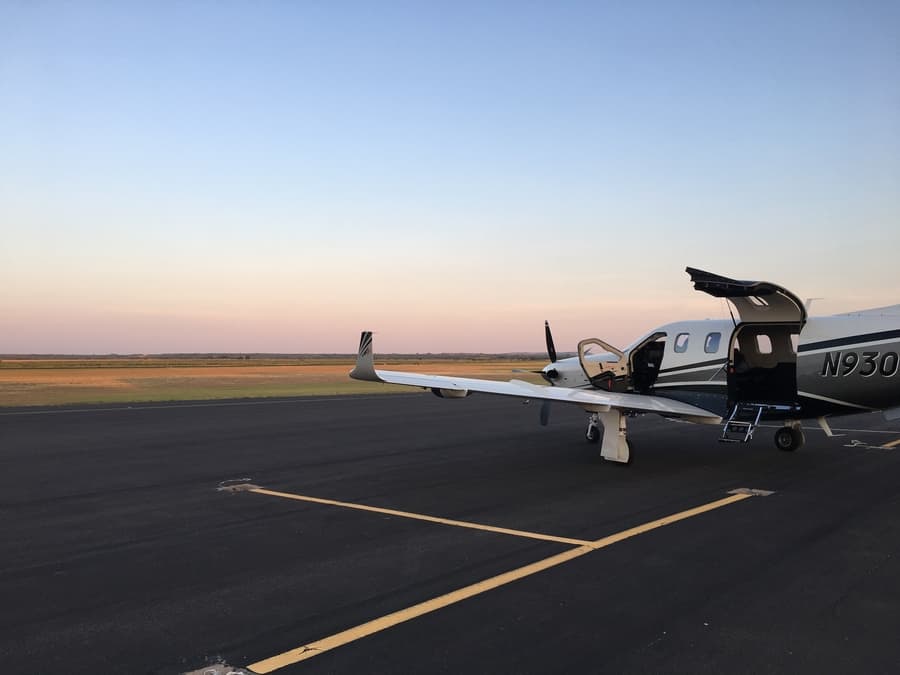
Piston and turboprop powered aircraft uniquely overlap in their flight regimes raising the inevitable question of which power plant is better. The two power sources can be compared in a range of categories, but this evaluation will focus on relative differences in safety, efficiency, cost, and performance. So what are the differences between piston and turboprop engines?
Piston engines are much more efficient at their typical power outputs and are less expensive both to purchase and operate. Turboprops are generally considered more reliable, offer higher efficiencies for their higher power outputs, and can yield much improved performance at high altitudes.
The advantages and disadvantages of each power plant are at times ill-defined and dependent on the mission of the owner or operator and on the intended flight regime of the aircraft, so it’s worth digging into the key differences of these engines from various angles to determine why they are best suited for particular operations.
This post is part of a three-part series comparing piston, turboprop, turbofan, and turbojet engines. You can see the other two posts here:
- Turbojet vs. Turbofan: Safety, Efficiency, and Performance
- Turboprop vs. Turbofan: Safety, Efficiency, and Performance
How Do Piston Engines and Turboprop Engines Work?
To inform the discussion of these two propulsion systems it is valuable to briefly review the operating principles of both piston and turboprops. Both turboprop engines and piston engines can be considered air pumps that extract energy from combustion to drive propellers.
How the engines achieve this is mechanically divergent but thermodynamically similar. As with all engines, there are four stages of the engine cycle: Intake, compression, combustion and exhaust.
How Piston Engines Work
Piston engines use fixed cylinders and reciprocating pistons linked to a common driveshaft to function.
In typical aircraft engines, each of the engine cycles occur on a separate stroke or rotation of the engine. This sequence is mechanically controlled by a camshaft that actuates valves at the top of each cylinder allowing the fuel/air mixture to enter the cylinder and be subsequently exhausted.
Virtually all piston engines used in aviation are direct drive, though a handful of geared engines have been placed into service.
Turbochargers and superchargers are used to increase the pressure of the intake air, effectively allowing the engine to operate at a lower altitude than the aircraft is actually at. Turbochargers are driven by exhaust gasses while superchargers are powered by the crankshaft of the engine.
How Turboprop Engines Work
Turboprops should be considered to be jet engines that drive a propeller using the exhaust gasses.
While piston engines have distinct divisions between the four engine cycles, turboprops seemingly blur the line between each. Air is drawn into the engine, where the compressor section increases pressure and temperature significantly, air is mixed with fuel and ignited simultaneously in the combustion section, while the turbine section controls the expansion of the exhaust and drives the propeller via a gearbox.
Power output is measured by torque and combustion temperatures. Many of these engines are considered “reverse flow” meaning that the intake air must reverse direction prior to entering the compressor, the PT6A is the most prolific example of this style of engine.
This video is a bit dated but provides a decent visualization of the turboprop engine:
For more reading on a few gauges and terminology specific to turboprop engines, see our article on torque, ITT, NP, and NG on turboprops explained.
Piston vs. Turboprop: Safety
Turbine engines have incredible reliability histories, and by extension, safety records.
The nature of turbine engines reduces or eliminates reciprocating mass, and thus vibration and fatigue factors that induce wear and tear are greatly reduced.
While a turboprop may have many more moving parts than a piston engine, those parts rotate in a single direction at relatively constant speeds with well-balanced mass. Additionally, there are virtually no components in turboprop engines that make physical contact with one another (gearboxes not withstanding). These factors all contribute to fantastic reliability and safety records.
The inherent reliability and safety of turboprop engines is why airlines and other high demand commercial operations that require consistent “up time” have migrated to the use of turbine engines, including turboprops.
As an example, Alaska Airlines uses the Q400, and FedEx uses Cessna Caravans for their respective short haul routes. These operations can depend on safe operation of their turboprops to deliver passengers and cargo, a testament and endorsement of turboprop safety.
Mechanically, turboprop engines offer safety advantages inherent to their design, and the rotating rather than reciprocating mass is only one advantage.
Turboprop engines generate significantly more excess air that can be used for ice protection systems, notably de-ice boots, providing safer operations in poor weather conditions.
Turboprop engines are also, in many cases, more tolerant of operator error, as the primary risks are over-torqueing the engine or exceeding ITT (Inter-Turbine Temperature) limits.
In comparison, pistons are at risk for overspeeding, detonation, cylinder overheating, improper mixture control, overboosted turbocharging systems, all as functions of operator error.
The simplicity of turboprop operations is one of their distinct safety advantages, particularly in higher performance, large, complex aircraft reduced workload is consistently a primary factor in improved safety.
The primary weakness of turboprops, mechanically, is the gearbox. The turbine components of the engine that deliver power to the propeller turn in excess of 30,000 RPM, while the propeller must be turned at speeds closer to 1,500 to 2,000 RPM.
Failure of this gearbox is the critical failure point of turboprop engines; the gearbox is protected by multiple propeller speed governors and is heavily lubricated. Modern turboprop installations include warning systems to detect the formation of metal shavings within the gearbox, allowing early detection of a failing engine.
Piston vs. Turboprop: Efficiency
Piston engines and turboprops are both internal combustion engines that must compress air, burn that air using fuel and expel the resulting exhaust gas. The thermodynamic properties that govern these engines result in interesting compromises between the two systems.
Generally speaking, turbine engines lose efficiency as they become smaller while piston engines become less efficient as they grow larger.
As an example, consider the following engines and their respective fuel flows at typical cruise power settings.
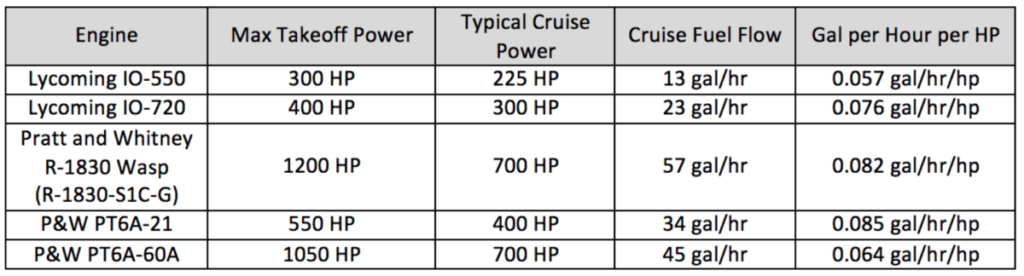
Before digging too deeply into the chart above, it is worth noting some details. The data above is representative, and non-specific to a particular aircraft, and represents typical values only.
Turboprop engine cruise data is more typically referenced with respect to torque output, but for the sake of direct comparison horsepower values are used.
Finally, the data above is for maximum continuous cruise, not for reduced power settings, this flight condition was selected to represent maximum performance of the engine.
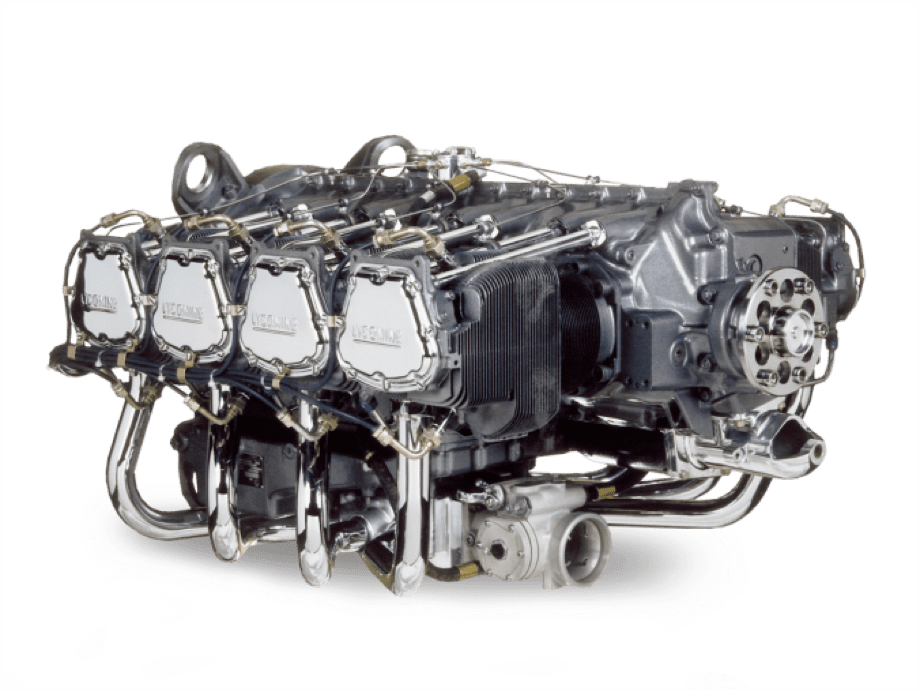
Source: Lycoming.com
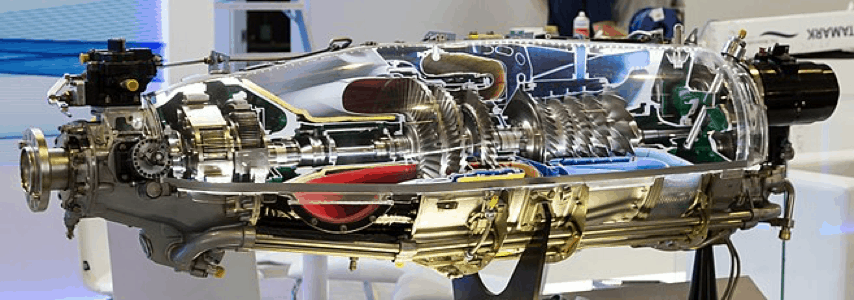
Source: Wikipedia Commons
Fuel Flow and Specific Fuel Consumption
Using a cursory review of the data above, the raw fuel flow values are misleading. The Lycoming engines appear to hold the advantage in terms of pure fuel flow, besting the PT6A-21 by at least 11 gallons per hour.
Closer inspection however reveals that the specific fuel consumption or Gal/Hr/HP value favors the IO-550 and the PT6A-60A. At the extremes of the power output ranges, efficiency is dramatically improved, the engines burn less fuel per hour to generate a given power output.
It is apparent that at some point larger and larger piston engines become less efficient relative to similarly sized turbine engines, and conversely, smaller turbines become less efficient than similarly sized pistons.
The exact crossover point where this happens is somewhat dependent on the particular installation and the mission profile of the aircraft.
This thermodynamic “quirk” is one reason why small turbine engines have not taken hold in the light aircraft market (purchase cost and installation weight being other reasons).
Turboprop Conversions
There are piston to turboprop conversions present in the light aircraft market, most notably the turbine Bonanza conversions as well as a Cessna 206 conversion. Both of these conversions serve as solutions to very narrow problems, Bonanza owners needing to fly higher than normal for water or mountain crossings and skydiver operators looking to maximize climb performance and descent rates.
This thermodynamic reality has driven aircraft designers toward selecting turboprop engines for single engine aircraft beginning in the 5,000 lb to 6,000 lb max takeoff weight range, as below this weight, 350 HP to 400 HP engines are sufficiently powerful to achieve sufficient takeoff and climb performance while remaining more efficient than small turboprops.
In terms of efficiency, pistons and turboprops find a crossover point in the 400 HP to 500 HP range. This thermodynamic fact limits the application of piston engines for large aircraft and the application of turboprops for small aircraft.
Pistons vs. Turboprops: Expense
Aircraft engines are, relative to the cost of their associated airframes, expensive; turboprops are certainly no exception. But cost goes beyond initial purchase price of the engine and its accessories, and extends to include maintenance issues that occur over the life of the engine.
Purchase Costs
New piston engines range in cost from $25,000 for small four cylinder engines to more than $100,000 for large six cylinder engines such as the Continental TSIO-550. The Pratt and Whitney PT6A series engines cost a minimum of $700,000 and easily exceed $1,000,000 for larger variants.
Clearly in terms of pure upfront cost, the piston engine is less expensive.
Turboprops have an advantage however in their required maintenance and overhaul schedules, which do benefit fleet operators that can closely track and manage engine maintenance intervals, are substantially longer and more predictable.
Overhaul and Maintenance Schedules
Piston engines have time between overhaul value ranging between 1,800 hours and 2,000 hours. Using the Continental TSIO-550 as an example with a TBO of 1,900 hours; the amortized cost of a new engine is ~$58/hour, while an overhaul (assuming a cost of $40,000) at TBO amortizes to ~$22/hour.
Most owners of piston aircraft elect to use an overhauled engine, when it becomes necessary to replace or overhaul the engine, and there is much to be said in regard to operating the engine beyond the specified overhaul interval. The typical challenge with piston engines, particularly high horsepower engines is that cylinders and cylinder heads often do not reach the full overhaul time specified.
It is not uncommon to see engines with disparate service times for various cylinders or “top overhauls” performed mid-lifecycle. New cylinders easily cost in the neighborhood of $1,000 each, making replacement unappealing but certainly economical relative to the cost of a new engine.
Turboprops in comparison have longer overhaul schedules and more costly overhauls as well.
Using the PT6A family of engines as an example, the baseline TBO is 3,600 hours, but depending on the aircraft, this may be extended to as long as 8,000 hours.
Amortizing the cost of a small PT6A with a $700,000 purchase price over a 3,600 hour TBO yields ~$195/hour, while an overhaul costing $250,000 costs ~$70/hour. With proper engine management and inspections, if this is extended to 8,000 hours, this cost drops further to ~$31/hour.
Turboprop operators benefit from more advanced maintenance regimens that are typically associated with the more complex and expensive aircraft they are generally installed in. These maintenance programs are designed to amortize the cost of complex and expensive maintenance across the life of the aircraft and its systems.
As such a direct comparison of operating costs due to maintenance between pistons and turboprops is difficult to conduct unless comparing directly to a specific operator with a specific maintenance and overhaul schedule.
Ultimately turboprop engines are more expensive to purchase and maintain, however, as discussed previously in relation to efficiency, turboprops are extremely cost effective relative to their performance and complexity.
The predictability and reliability of turboprop engines allow fleet operators to closely plan the cost of operation down to the week or even day, making financial performance planning much easier.
Piston engines can achieve some of this predictability, but ultimately due to the mechanical components of piston engines, they present a substantially more challenging maintenance prediction problem.
Fuel Costs
Finally, discussion of cost would be incomplete without a discussion of fuel costs. Referencing AirNav.com average fuel price data as of December 2019, 100LL fuel (avgas) averages $5.06/gallon and Jet-A averages $4.68/gallon (see our article on “Why does jet fuel cost less than 100LL?”).
Applying these averages to the fuel burn data above in table 1 the following direct fuel costs result:
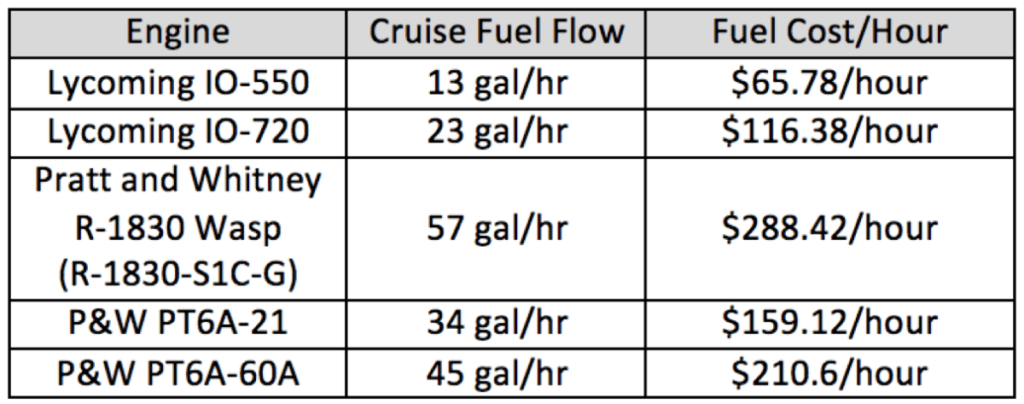
It is again evident that the turboprop engines command a premium with regard to direct fuel costs at the “low” end of the power range; however, that margin narrows as the power output of the turboprop engines increases.
This is a direct result of the improved thermal efficiency of turboprop engines. This fact again makes the operating costs of small turboprop engines difficult to justify in small aircraft such as the Beechcraft Bonanza or the Cessna 206 Stationair.
Ultimately for both types of engines, operating cost is managed by good engine operating habits and by proper maintenance and inspection intervals.
The most reliable piston engines and most reliable turboprop engines are those that are properly managed as the complex and expensive equipment that they are. While piston engines have a greater number of direct wear parts, it is generally simpler and less expensive to service those wear parts.
Replacing a cam or a valve is generally a straight forward exercise for a piston engine, while accessing and replacing a turbine blade is substantially more difficult and expensive proposition in a turboprop engine.
Piston vs. Turboprop: Performance
Piston engine performance and turboprop performance, while similar in some respects, is an illustration of the benefits of turbine engine operating characteristics. The performance envelope of a given engine is best described by cruise performance and takeoff performance.
In the case of both piston and turboprop engines, total power output is measured in horsepower, and the engine will be capable of generating maximum continuous power up to a “critical altitude;” above which, performance is reduced.
Performance of Piston Engines
In order to understand the performance characteristics of piston and turboprop engines it is necessary to review the basic operating characteristics of each.
For normally aspirated piston engines, maximum continuous power output is typically 75% of the maximum rated power; most piston engines will maintain this power output until approximately 7,000 feet to 9,000 feet pressure altitude.
Turbocharging can improve this altitude up into the teens, or even the lower flight levels.
The Cessna 182 Turbo has a critical altitude of 20,000 feet, the same as its service ceiling, the Cessna T206H Turbo Stationair maintains sea level power to 16,000 feet while its service ceiling is FL240, and the Beechcraft Baron BE58TC has a critical altitude of approximately FL180 with a service ceiling of FL250.
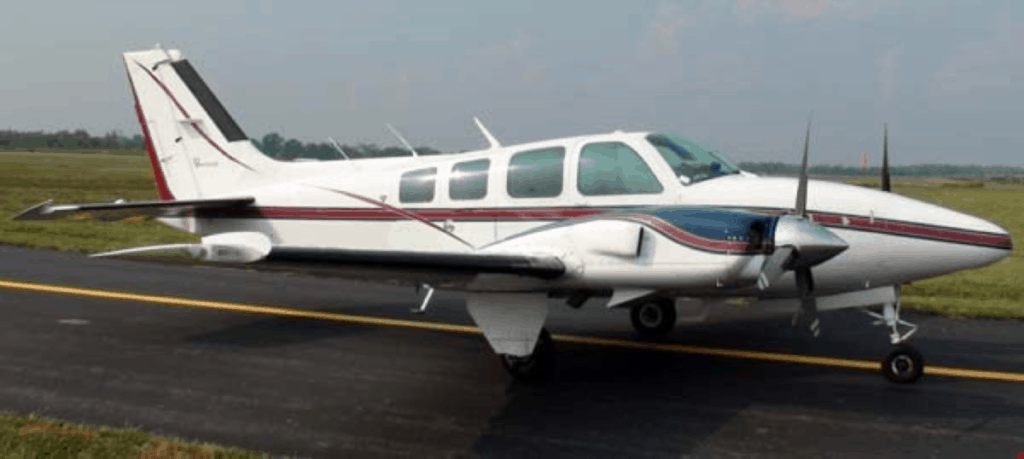
Source: GlobalAir.com
Obviously turbocharging can yield significant performance improvements in cruise. The Baron achieves cruise speeds well above 200 knots as a result of its ability to maintain high power output to very high altitudes.
Modern piston powered aircraft are generally limited to cruise speeds in the 200-230 knot range with a few experimental aircraft reaching speeds approaching 300 knots. Achieving cruise speeds above the 230 knot range becomes difficult relative to the performance capability of a turboprop.
Piston takeoff performance (assuming a constant weight and pressure altitude) is a function of the power output available, again a function of the engine’s critical altitude.
For a given pressure altitude the aircraft’s propeller and wings will achieve whatever level of efficiency and performance is available to them at that altitude, meanwhile the ability of the engine to generate as much power as it can provides sufficient energy to become airborne using whatever amount of air is available.
The result is normally aspirated engines see a greater takeoff performance reduction at high altitudes than their turbocharged siblings, but both normally aspirated and turbocharged piston aircraft have reduced takeoff performance at high altitudes.
Performance of Turboprop Engines
Turboprop powered aircraft face similar performance impacts as pistons, the basic impacts of atmospheric density and pressure apply equally. However, because turboprops benefit from both higher baseline power outputs and more efficient power generation, turboprops are able to perform at levels only dreamed of by piston pilots.
Considering cruise performance, turboprops also have a critical altitude, it is defined by when the engine becomes limited by the ITT (Inter-Turbine Temperature) rather than by torque output.
At takeoff from sea level a turboprop will be cable of making its maximum rated torque below its maximum temperature limit, but as the aircraft climbs the ITT will also rise (the piston equivalent of leaning the mixture) reaching some maximum continuous value, this is the critical altitude for a turboprop. This altitude varies widely across the range of engines, even across engines with similar power outputs.
There are hosts of engine and aircraft design reasons for this inconsistency, the critical altitude is a function of the margin between the ITT at which the engine achieves its rated power and the maximum ITT the engine components can sustain.
Aircraft manufacturers may elect to “de-rate” an engine, essentially limiting its torque output to achieve greater ITT margin, and thus an artificially higher critical altitude.
For example, the Cessna Caravan with the 675 horsepower PT6A and the Caravan EX with the 867 horsepower PT6A both have critical altitudes in the 7,000 foot to 8,000 foot range depending on temperature, but the Caravan EX will climb well above the 675 Caravan and will maintain better airspeeds at higher altitudes as a result of the raw power improvement.
As a contrast to the Caravan, the King Air 350 maintains maximum torque to 16,000 feet, and the King Air 250 maintains maximum to torque to approximately 5,000 feet.
Continuing the examination of the King Air 350, its engines allow it to achieve cruise altitudes as high as FL350 while maintaining true airspeeds in excess of 250 knots. This high-altitude performance is the primary advantage of turboprops.
The ability to climb over most weather while maintaining reasonable cruise speeds, while maximizing efficiency, gives distinct advantages when planning long distance flights.
When maximum efficiency or weather avoidance is not a primary consideration, the 350 is able to achieve 300 knot cruise speeds at altitudes similar to the BE58TC, easily a 70 knot advantage, while carrying significantly more passengers or cargo, all in pressurized, air conditioned comfort.
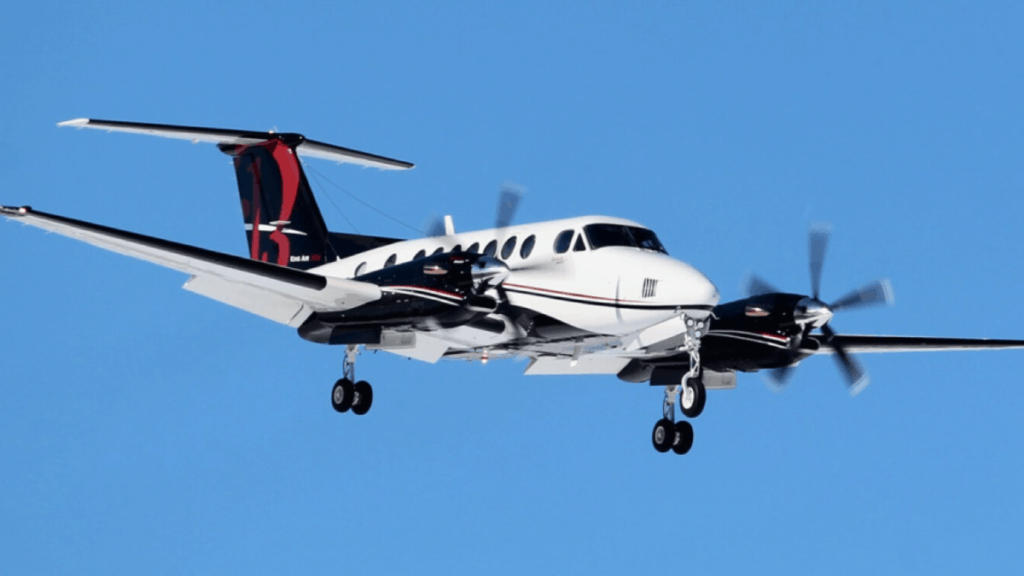
Source: ChronoAviation.com
It is unreasonable to directly compare turboprop and piston takeoff distances between aircraft with widely divergent takeoff weights.
Comparing Piper’s M350 and M500, which are similar airframes, the M350 with a Lycoming TIO-540 and the M500 with a PT6A-42A, reveals a 700 pound weight increase on the M500. The claimed takeoff performance for the M500 shows a 350 foot increase in takeoff distance.
However, the M500 does claim a 50 knot improvement on cruise speed. In essence turboprop engines do not radically improve takeoff distances for equal power outputs, as the propeller is still transmitting the same output to the surrounding air.
The advantage of turboprops is the ability to maintain that power at higher altitudes.
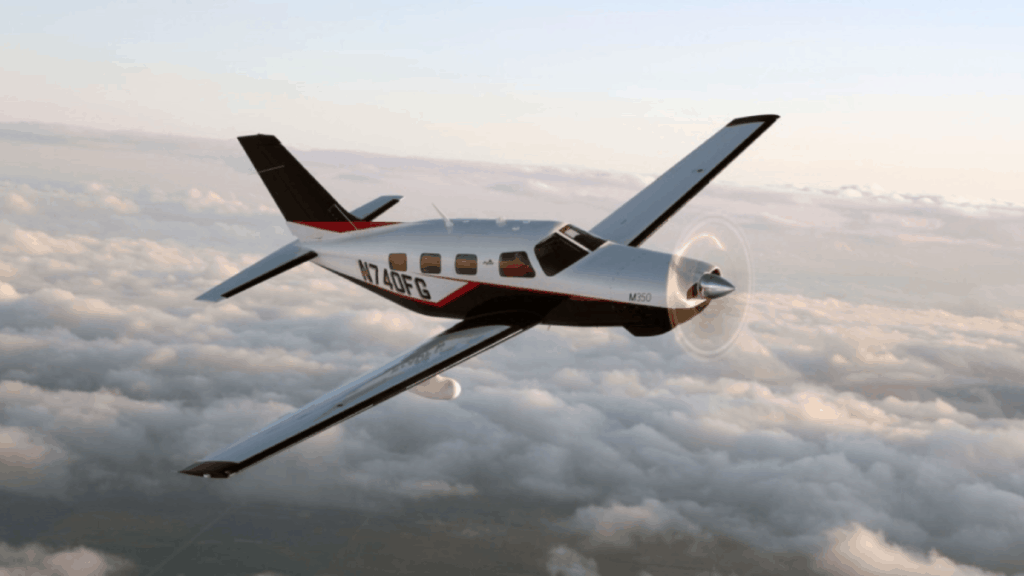
Source: Piper.com
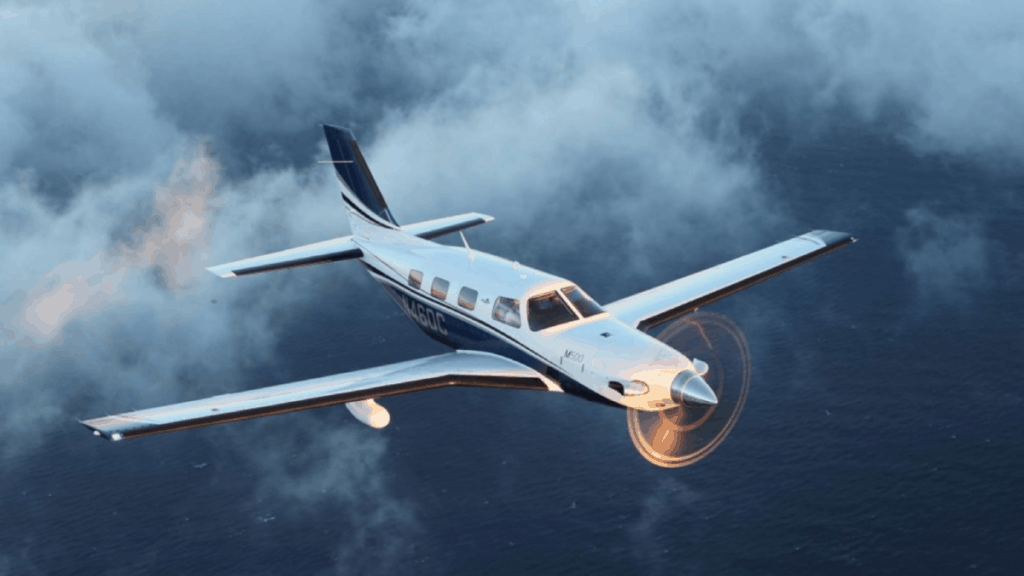
Source: Piper.com
Turboprops do gain an advantage during landings; the reversing and feathering propellers installed on turboprops dramatically improve landing performance relative to their weight.
Comparing the previous example of the M350 and M500, the 700 pound heavier M500 sees only a 142 foot increase in landing distance.
It is worth noting that typical turboprop certification does not provide credit for reverse thrust, only “beta” that is a propeller that achieves neutral thrust output by moving the blades to be perpendicular to the incoming flow. Use of full reverse may further improve this distance, in the practical world.
Piston vs. Turboprop: Performance Overview
The performance advantages and disadvantages between turboprops and pistons are, as with most aviation design decisions, a function of the intended mission.
If the intended mission of the aircraft is to fly above weather and at higher true airspeeds, turboprops quickly become very attractive solutions. The ability to maintain better power output and the inherent efficiency of higher output turboprop engines is well worth the relative penalty in weight or fuel flow.
By comparison piston engines become performance limited at lower altitudes, and without complex and heavy turbocharging systems are largely relegated to the low teens.
The transition to turboprops has been driven by the need to improve the efficiency; speed and altitude of aircraft that need to achieve long range missions or carry large, heavy loads.
Conclusion
The safety, efficiency, cost and performance advantages and disadvantages of pistons and turboprops are a function of the aircraft’s weight, and engine’s power output.
Small turboprops become too heavy and inefficient relative to their power output, but large piston engines are too complex and difficult to manage relative to their power output.
Each engine class has applications that best suit their strengths.
Turboprops are outright “better” from a safety perspective, but otherwise each type of engine is best considered as optimized for a specific class of aircraft. Extremely large piston engines compare poorly to similar output turboprops, but small turboprops eventually lose out to piston engines.
As with most things aviation, each engine type is a compromise of attributes that serve to best achieve the intended mission of the aircraft.